ARTICLE AD
The resting mass of ghostly neutrinos is one of the most sought-after quantities in particle physics that scientists are one step closer to accurately determining, thanks to a new experiment led by researchers from the Max Planck Institute for Nuclear Physics in Germany.
If the mass of neutrinos was known, it could crack open the door towards physics beyond the realm of the Standard Model of particle physics, which describes all known forces and elementary particles in the Universe.
To say neutrinos are weird is an understatement. Once suggested to have no mass at all, it's now clear this barely-existing particle is actually three-types-in-one, with identities that oscillate in a strange quantum blur as they zip through space. This flickering identity implies mass, which also comes in different forms, smeared across the neutrino's shifting guise.
Being so light, and strange, neutrinos may not play by the same rules as other particles. Adding up the smear of its incredibly small masses with precision may even help confirm and rule out new models in particle physics.
Physicists can't simply weigh bunches of stationary neutrinos like grapes sitting on a scale, however. Instead, they can only confirm the presence of such subatomic particles by looking at their interactions with other particles, or by measuring the products of their decay. A particle might only be present for the briefest moment but in that time, it leaves its mark or trace from which physicists can infer mass.
However, with no charge and virtually no gravitational pull, neutrinos exert only the faintest of forces on other particles. In fact, billions of neutrinos are zipping through your body right now, most en route from the Sun, but rarely do they interact with us.
Yet just because they have so little sway over other subatomic particles doesn't mean neutrinos aren't a fundamental part of what makes up matter. They're the most abundant particles that have mass in the Universe, and knowing what gives those variations of neutrino such tiny, non-zero resting masses could help physicists iron out or understand some of the contradictions in the Standard Model that neutrinos present in the way they oscillate.
Physicists have been steadily refining their best estimates of the upper limits of neutrino's individual and collective masses using various methods. The most precise measurement yet of a 'flavor' called the electron neutrino found it couldn't be more than 0.8 electronvolts. Translating that to mass relative to 1 kilogram (or 2.2 pounds), it's like the weight of four raisins compared to the Sun.
Set in February 2022, the latest estimate was produced by the Karlsruhe Tritium Neutrino Experiment (KATRIN) in Germany, inferred from the spray of electrons and neutrinos released as a superheavy form of hydrogen decays.
Another way to get at the mass of a neutrino, however slight, is to study what happens when the atomic nucleus of an artificial isotope holmium-163 absorbs an electron from its innermost shell. As a result, a proton is converted into a neutron, the element dysprosium-163 is produced, and a neutrino is released.
Physicists can then measure the total energy released in this decay using a calorimeter of sorts, and infer the mass of the 'missing' neutrino that flew off into the ether based on the total mass of the atom and Einstein's famed equation, E = mc2, where mass and energy are equivalent.
This is calculated as what's called the Q value: a difference in energy which can be translated into the mass 'missing' from the sum total of atomic particles after a decay reaction. That difference in mass is interpreted as the neutrino.
However, the gold atoms in which holmium-163 is embedded could influence this decay reaction, explains Christoph Schweiger, a physicist at the Max Planck Institute for Nuclear Physics and lead author of the new study.
"It is therefore important to measure the value of Q as precisely as possible using an alternative method and to compare it with the calorimetrically determined value in order to detect possible systematic sources of error," he says.
To do that, Schweiger and colleagues set up an experiment that combined five so-called Penning traps, stacked one on top of the other inside a superconducting magnet placed in a vacuum and immersed in liquid helium at about 4 degrees Kelvin (-269.1°C or -452.5°F).
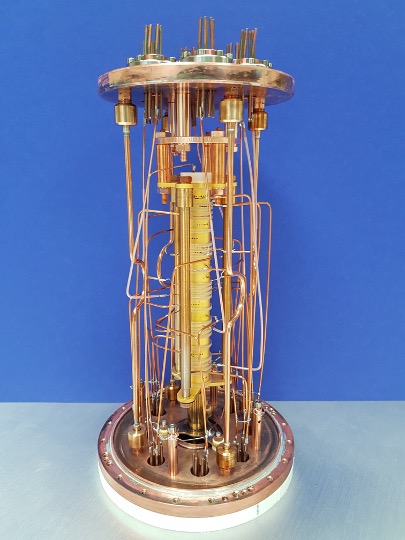
All that effort helps shield the equipment so that it's sensitive enough to catch particles in its Penning traps and measure the subtle energy differences between charged holmium-163 and dysprosium-163 ions.
"With an Airbus A-380 with a maximum load, you could use this sensitivity to determine whether a single drop of water has landed on it," Schweiger says.
In actuality, the researchers measured the inbound holmium-163 ions and resulting dysprosium-163 ions to come up with a Q value of 2,863.2 ± 0.6 eV c−2, which is 50 times more precise than a previous effort that arrived at a value of 2,833 ± 34 eV c−2.
Using a more precise and independently measured Q value in conjunction with other experimental results "is vital for the assessment of systematic uncertainties in the neutrino mass determination," Schweiger and colleagues write in their published paper.
Though just one piece of the puzzle, improved precision in measures like Q can be combined with the wide variety of approaches in understanding why the weird, shimmering ghosts of the particle world behave like the poltergeists they are.
The study has been published in Nature Physics.